Written by Logan Morrison Edited by Dr. Hayley McLoughlin
Research group uncovers the key molecular interaction that causes spinocerebellar ataxia type 1 (SCA1).
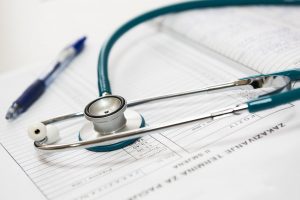
When we talk and think about human disease, it is natural to focus on causes. For some disorders, the source of the problem is clear: there’s no question why a patient with a spinal cord injury has paralysis, for instance. Other diseases, like schizophrenia, are incredibly difficult to attribute to specific environmental influences or genetic mutations (probably because they are the result of a variety of subtle factors that add up to cause the disorder).
Our current understanding of spinocerebellar ataxia type 1 (SCA1) falls somewhere in between these extremes. For years, we have known that SCA1 is caused by a polyglutamine expansion in the ataxin-1 gene. In short, this means that SCA1 patients have experienced a rare copying error in their genetic code in the region that is responsible for guiding the production of the Ataxin-1 protein (ATXN1). However, there are still quite a few questions surrounding what ATXN1 does under normal circumstances. This has meant that, so far, scientists have not been able to show why a polyglutamine expansion in the ataxin-1 gene causes the cells of the cerebellum, spine, and brainstem to lose their normal function in cases of SCA1.
This all changed in March 2018, when the labs of Dr. Harry Orr (University of Minnesota) and Dr. Huda Zoghbi (Baylor College of Medicine) published a monumental paper in the journal Neuron1. This study is a continuation of the fantastic collaborative research between Drs. Orr and Zoghbi, whose working relationship goes all the way back to 1993 when the two researchers discovered the mutation that causes SCA1 on the same day2. The strides they have made in the SCA field have highlighted how disease research is truly a team effort – a point made especially clear by the fact that this current study was published with 21 different authors.
In this study, Orr and Zoghbi aimed to tackle a problem that has challenged SCA1 researchers for quite some time. When a mutation in a gene is the known cause of a disease, it makes sense to assume that the mutation has disrupted the gene in a negative way – impairing its normal function. And, in the majority of genetic diseases, this is precisely what happens3. However, in a previous study of SCA1, mice were genetically engineered to produce no ATXN1 in the brain at all. These animals, surprisingly, showed no symptoms of ataxia (though they did have some other problems, such as a learning deficit)4. This meant that the SCA1-causing mutation doesn’t simply impair the function of ATXN1 – it does something else entirely. Nearly 20 years after this puzzling result, Orr and Zoghbi set out in this study to determine what exactly that “something else” is.
There has been strong evidence in the past that ATXN1 interacts with another molecular machine, capicua, in the cells of the brain, and that this interaction could very well play a role in the pathology of SCA15-7. To investigate this further, the team genetically engineered a line of mice with a mutation that would target the interaction site of ATXN1 and capicua, specifically in the cerebellum. They then bred these mice with a mouse line that carried the SCA1-causing mutation. The offspring of this mating, then, would have the SCA1-causing mutation, but their mutated ATXN1 would be incapable of interacting with capicua in the cerebellum.
Remarkably, as these mouse pups grew to adulthood, they seemed to have very little evidence of SCA1 pathology. In a typical SCA1 mouse, movement symptoms are hard to miss, especially in motor performance tests like the rotarod. Think of the rotarod as a log-rolling challenge for mice: the animals are placed on a small rotating cylinder, and work to stay on for as long as possible. This measures balance, coordination, and grip strength, and has been used for decades as an indicator of an animal’s fine motor skills – mice with the SCA1 mutation, for instance, can only stay on the rotarod for half the time a normal mouse can. However, as these researchers found, the SCA1 mice had perfectly normal rotarod performance when their ATXN1 was unable to interact with capicua. They also found that these mice had no significant cell death and/or decay in their cerebellum once they reached adulthood, unlike the typical SCA1 mice. And, to top it all off, Orr and Zoghbi link their mouse model directly to human patients. By comparing cells from SCA1 patients with cells from their unaffected siblings, they discovered that a subset of genes were more active when the “diseased” ATXN1 was present. Many of these genes – downstream targets of capicua – also showed increased activation in the authors’ mouse model. Overall, this shows that the ataxin-1 mutation seen in patients seems to be causing SCA1 due solely to its interaction with capicua.
This could be great news for SCA1 patients, mainly due to the nature of drug development. As the pharmaceutical industry has grown over the years, we have found that it is usually much easier to inhibit a specific chemical process in the body than to activate it3. This can be seen when we look at many of the most common drugs in America, such as ibuprofen, atorvastatin (i.e., Lipitor), omeprazole (i.e., Prilosec), lisinopril (i.e., Prinivil), and acetaminophen – all of which work because they decrease the function of certain proteins in our body8. Now that we know it is a single protein interaction that causes SCA1 pathology, the prospect of creating a drug to target the ATXN1-capicua binding site sounds like it could be a turning point in the treatment of this disease. Unfortunately, blocking the specific interaction of two proteins is not as simple as just inhibiting a single protein, like Lipitor does; however, research in this field is coming of age, and recent advances have provided hope in the fight against heart disease, cancer, and HIV using drugs that target protein-protein interactions9, 10. At any rate, what Orr and Zoghbi have revealed with these experiments represents an incredible step forward in our understanding of SCA1, solving a genetic mystery that has plagued the scientific community for decades.
Key Terms
Polyglutamine Expansion Disorder: In about 60 of our genes, there is a DNA code that instructs cells to make small molecular machines (known as proteins) that have large, repeating chemical structures. These structures are made up of the chemical glutamine. While glutamine repeats serve a valuable function under normal conditions, they can also be quite unstable. In polyglutamine expansion disorders, the process of copying these repeating DNA codes becomes disrupted sometime early in development, causing an out-of-control expansion of the number of glutamines that a protein is supposed to contain. Because each gene has a different function, the gene in which a polyglutamine expansion occurs determines which disease a patient has – for example, SCA1 occurs from a polyglutamine expansion in the ATXN1 gene, while Huntington’s disease occurs from a polyglutamine expansion in the IT15 gene.
ATXN1: Ataxin-1 (abbreviated as ATXN1) is a small molecular machine known as a protein. Its function is to guide the creation of other proteins from our DNA blueprint, making it an incredibly important indirect player in a variety of cellular processes. When the gene encoding ATXN1 undergoes a rare mutation that elongates the resulting protein, the result is SCA1.
Cerebellum: A primary area of pathology in the spinocerebellar ataxias. This brain region sits toward the back of the skull and, though small in stature, contains the majority of the nerve cells (neurons) in the central nervous system. Contains the circuits that fine-tune our movements, giving us the ability to move with precision.
Brainstem: The connection between the spinal cord and the brain. Among the many connecting fibers in this brain region, there are a host of processing centers for our most basic physiology: breathing, heart rate, etc. In SCAs, the brainstem experiences degeneration later in disease progression, which is thought to be the cause of premature death in patients.
Rotarod: The rotarod is a technique that has been used for decades to test motor performance in mice and rats. Researchers place the animal on a rotating cylinder and record how long it takes for the animals to fall off as the rotation speed is gradually increased. The higher the mouse/rat’s motor performance, the longer they will be able to stay on.
Conflict of Interest Statement
Logan Morrison, who wrote this piece, is currently doing his graduate work in Vikram Shakkottai’s lab, where he is working on a project in collaboration with Dr. Harry Orr. Dr. Hayley McLoughlin, the editor of this piece, has no conflicts of interest to declare.
Citation of Article Reviewed
Rousseaux, M.W.C., et al., ATXN1-CIC Complex Is the Primary Driver of Cerebellar Pathology in Spinocerebellar Ataxia Type 1 through a Gain-of-Function Mechanism. Neuron, 2018. 97(6): p. 1235-1243 e5. (https://www.ncbi.nlm.nih.gov/pubmed/29526553)
References
- Rousseaux, M.W.C., et al., ATXN1-CIC Complex Is the Primary Driver of Cerebellar Pathology in Spinocerebellar Ataxia Type 1 through a Gain-of-Function Mechanism. Neuron, 2018. 97(6): p. 1235-1243 e5.
- Chiaet, J., Neuroscientist Who Doggedly Pursued Genetic Hunch Wins Pearl Meister Greengard Prize, in Scientific American. 2013.
- Chen, B. and R.B. Altman, Opportunities for developing therapies for rare genetic diseases: focus on gain-of-function and allostery. Orphanet J Rare Dis, 2017. 12(1): p. 61.
- Matilla, A., et al., Mice lacking ataxin-1 display learning deficits and decreased hippocampal paired-pulse facilitation. J Neurosci, 1998. 18(14): p. 5508-16.
- Lam, Y.C., et al., ATAXIN-1 interacts with the repressor Capicua in its native complex to cause SCA1 neuropathology. Cell, 2006. 127(7): p. 1335-47.
- Fryer, J.D., et al., Exercise and genetic rescue of SCA1 via the transcriptional repressor Capicua. Science, 2011. 334(6056): p. 690-3.
- Kim, E., et al., Structural basis of protein complex formation and reconfiguration by polyglutamine disease protein Ataxin-1 and Capicua. Genes Dev, 2013. 27(6): p. 590-5.
- Law, V., et al., DrugBank 4.0: shedding new light on drug metabolism. Nucleic Acids Res, 2014. 42(Database issue): p. D1091-7.
- Arkin, M.R., Y. Tang, and J.A. Wells, Small-molecule inhibitors of protein-protein interactions: progressing toward the reality. Chem Biol, 2014. 21(9): p. 1102-14.
- Zhan, P., et al., Targeting protein-protein interactions: a promising avenue of anti-HIV drug discovery. Curr Med Chem, 2010. 17(29): p. 3393-409.