At NAF, we support researchers in many ways, including our grant process. Research grants allow Ataxia investigators to request funding for their studies. Lay summaries are available below for the research grants we funded in 2021.
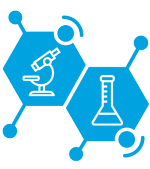
$655,000 Funded Overall
13 Research Studies Funded
Research Seed Money Grants
Research Seed Money Grants are available for new and innovative studies that are relevant to the cause, pathogenesis or treatment of the hereditary or sporadic Ataxias. This type of grant is offered primarily as “seed monies” to assist investigators in the early or pilot phase of their studies and as additional support for ongoing investigations on demonstration of need.
Click the carrot next to the name of the study to see its summary.
Nadia D’Ambrosi, PhD
University of Rome Tor Vergata, Department of Biology
Rome, Italy
Role of iron-dependent dysfunctions in microglia toxicity*
Summary:
It is widely recognized that the nervous system is a primary target in Friedreich’s ataxia (FRDA), with specific brain and spinal cord regions displaying significant atrophy and degeneration. Neuron loss is usually a permanent event, since nerve cells are poorly replaced by adult stem cells, leading therefore to derangement of brain areas, with dramatic consequences. In most diseases characterized by nerve cells injury, neuron fate relies not only on intrinsic pathological mechanisms, rather neuron loss is often preceded and accompanied by activation of neighboring non-neuronal cells, that contribute to establish the neuronal outcome of these diseases. Microglia, in particular, are considered the immune cells of the central nervous system, where they represent 10% of the total cell population. They constantly surveil the extracellular environment and respond to multiple stimuli, in order to kill and remove possible harmful agents. Malfunctioning neurons are a source of signals that activate adverse responses by microglia, culminating into further damage to neurons, recognized by microglia as undesirable elements that have to be removed. Nevertheless, under certain circumstances, microglia can also exert trophic and pro-survival actions that can support neuron integrity. Therefore, the possibility to switch microglia towards this beneficial side can be of advantage. The multifaceted role of microglia is well defined in neurodegenerative conditions such as Alzheimer’s and Lou Gehrig’s, as well as fronto-temporal dementia, where the replacing of harmful microglia with a beneficial type has provided many encouraging results in slowing-down the progression of the diseases. In FRDA, there is a limited knowledge about the toxic functions assumed by microglia as a consequence of frataxin-dependent iron accumulation. However, important papers published in very recent years, demonstrated that reactive microglia populate affected brain areas and that the transplantation of healthy microglia protects to a certain extent tissue damage in FRDA mice models. These data provide a robust indication that microglia can contribute to neuronal demise also in FRDA. With this project, we aim to obtain a direct demonstration that FRDA-related pathological mechanisms encompass the neurotoxic conversion of microglia. To this purpose, we will employ microglia derived from healthy and FRDA affected mice to dissect how iron accumulation, derived by frataxin loss-of-function, drives incorrect mechanisms in microglia that alter their mitochondria, their production of damaging free oxygen radicals and their release of pro-inflammatory molecules, transforming them into possible neurons’ foes. Finally, we will directly demonstrate how microglia from FRDA mice impair neuron survival and thus how these cells contribute to neuron demise in the disease. The clear identification of microglia as main players in the pathology will open new venues for a possible treatment strategy. The understanding of microglia function in the disease obtained by the expected results of this project could help in developing therapeutics, which take into account and match the specific functional states microglia, in order to drive them towards a neuron protective state. Under this aspect, it is conceivable that halting a complex and multi-systemic disease as Friedreich’s ataxia should require a multitargeted approach. With this research project, we will identify microglia toxic activation as a novel mechanism implicated in FRDA pathogenesis, indicating new potential therapeutic strategies to be exploited to counteract the disease.
*Co-funded in collaboration with FARA
Khalid El Hachimi, PhD
Institut du Cerveau et de la Moelle epiniere
Paris, France
Role of KIF1C motor protein in myelination – Involvment in ataxia
Summary:
Persistent ataxia is a neurological sign result from brain damage. This peculiar damage affects the brain centers that controls voluntary muscle movements and coordination. These movements disorders induce also difficulties in speech, eye coordination movements and swallowing. The cause of the persistent ataxia are diverse; toxic, metabolic, genetic or both. Our laboratory, located at Salpêtrière hospital (Paris) which include the largest neurological diseases care department in France, is involved in the research of genetic causes of the ataxia. The team is leaded by Alexdra Durr and Giovanni Stevanin. We have collaborated in the identification of several forms of genetically determined ataxia. We also studied the biological mechanisms underlying these peculiar forms of ataxia and developed preclinical therapeutic approach against this disease assocaited to this clinical sign. Recently, we and others have shown that autosomal-recessive mutations in the human gene coding for a motor protein belonging to Kinesin protein family KIF1C lead to neurodegenerative spastic ataxia. Soon after, we discovered that a homozygous mutation in the bovine KIF1C gene is the leading cause of progressive ataxia in Charolais cattle. The biological role of KIF1C in the CNS and specially the pathomechanistic link between KIF1C deficiency and cerebral/cerebellar dysfunction in spastic ataxia, remains however, poorly understood. Amandine Duchesne researcher at the “Institut national en recherches agronomiques et écologiques” (INRAE) and myself generated a mouse model mimicking clinical and pathological features of this peculiar ataxia (SAX2/spastic paraplegia58). We are also and currently developping a novel V5-tagged Kif1c model mice which allow us to identify the RNA and protein parteners of KIF1C. Our integrative scientific approach is based on collaboration with physicians and researchers from different disciplines. For that we have biological samples (cattle and mice) to perform all the studies. We have developed at our institute (Paris Brain institute, ICM) many tools (behavioral, radiological, histopathology, and molecular) to carry out this study. We have a performant facilities in rodent phenotyping (Phenoparc), small animals neuroradiological investigations (CENIRS), neuropathological characterization (Histomics), cell culture and cellular imaging (Quant, Celis)) and molecular analyzing. Within the institute we have also developed very good technical skills. These mice models, for which we request financial assistance from the National Ataxia Foundation, may allows us to decipher the biological aspect of the cerebellum dysfunction and to propose rational approach for preclinical therapeutic strategy, before their hopefull extention to man. We hope that our study draws your attention, worth being considering by scientific board, and we would be happy to be encouraged by the National Ataxia Foundation.
Andreia Teixeira-Castro, PhD
Life and Health Sciences Research Institute (ICVS) and ICVS/3B’s – PT Government Associate Laboratory, University of Minho
Braga, Portugal
Intermittent fasting as a novel therapeutic strategy for SCAs
Summary:
Spinocerebellar ataxias (SCAs) are diseases of the nervous system, in which certain neurons, including neurons of the cerebellum, the motor coordination center of the brain, slowly degenerate. Common symptoms of ataxias are lack of coordination, slurred speech, trouble with eating and swallowing and deterioration of fine motor skills. Extensive research has been conducted to understand how the disease occurs and address the disease causes. Spinocerebellar Ataxia type 3 (SCA3) or Machado–Joseph disease (MJD) is the most frequent ataxia worldwide, and affected individuals have a 50% chance of transmitting the affected gene to their children. In SCA3, a mutation in the ATXN3 gene generates a bigger-than-normal size of polyglutamine tract of ATXN3 protein, causing neurons to die and ultimately leading to the patients’ death. Existing treatments for SCA3 are very limited, only improving symptoms, and not stopping disease progression, making the search for new therapeutics an urgent need. The primary goal of aging research is to improve the health of the elderly and to discover ways of stopping or delaying age-related diseases. Several factors like genetics, socioeconomic status, environmental quality, and alimentary habits affect how long and how healthy people live. Although genetics and environmental quality are not under our direct control, alimentary habits, especially the amount and quality of food are. Proper nutrition can influence health and survival and often delay or prevent the start and progression of diseases. However, eating too much or too little has been associated with a high risk of chronic diseases and early death. Importantly, in our modern society, eating too much is a major risk for several diseases. A nutritionally balanced diet based on alteration of caloric intake or meal timing can lead to disease control and a healthier and longer life. There are four main diets that have been proposed to improve health: caloric restriction, in which people eat less calories per day; time restricted feeding, in which people eat only during certain hours of the day; alternate-day fasting, in which people fast during fixed periods each week; and fasting-mimicking diets, in which people eat only certain food types that mimic a fasting-like state in the body- the ketogenic diet. The classical caloric restriction was the first type of dietary regimen to be widely used for disease intervention and, only many years after, it was discovered how caloric restriction might improve disease progression: because eating less calories is often accompanied by long fasting periods, our bodies stop using sugar (glucose) as a fuel source, using ketones instead- the so-called metabolic switch that leads to many neuroprotective features. It is likely that more people may adopt these eating habits, because, compared to caloric restriction, these diets are less difficult, in the long-term. In this study, we will test different diets as a therapeutic strategy for SCA3 in rodents. Without any reduction in caloric intake, a metabolic switch will be induced by daily limiting feeding periods to certain times of the day, and by feeding the animals with a fast-mimicking ketogenic diet. We will test if these different alimentary habits stall disease progression in mice. If these diets show an impact on SCA3 animals, this could be relevant also for other ataxias.
Marka Van Blitterswijk, MD, PhD
Mayo Clinic Jacksonville
Jacksonville, FL
Long-read sequencing to decipher a repeat expansion causative of SCA36
Summary:
Spinocerebellar ataxia 36 (SCA36) is a condition characterized by problems with balance and coordination resulting from the progressive atrophy of various regions of the brain, in particular the cerebellum. The cerebellum receives information from the spinal cord and other parts of the brain, and uses this information to coordinate voluntary movements. In addition to balance and coordination problems, people with SCA36 may also experience exaggerated reflexes, difficulty with speech, tongue muscle twitches and wasting, and eventually muscle atrophy in the legs, forearms and hands. The cause of SCA36 is a longer than usual repetitive DNA sequence in a gene called NOP56. We seek to untangle how this repetitive sequence gives rise to SCA36. To this end, we will use a highly innovative technology called No-Amp sequencing. This technology enables us to perform in-depth studies focusing on the repetitive area in NOP56. We can accurately assess how long the repetitive stretch is and whether there is variability in its length across and within patients. Moreover, we will evaluate the content of the repetitive sequence itself. Although it has been reported to contain a stretch of GGCCTG-repeats, it has yet to be determined whether it is pure or interrupted by other sequences. Additionally, we will investigate whether the repeat is methylated and, if so, to what extent. Thus, our original approach – to examine the entire span of the repetitive sequence using cutting-edge technology – gives us the opportunity to reveal whether its length, purity and/or methylation profile may modify the symptoms or severity of SCA36. Furthermore, we will examine whether these characteristics of the repetitive sequence itself influence crucial disease-associated features of SCA36, such as the expression levels of NOP56 and the production of faulty RNAs or abnormal proteins. Hence, our proposed project will aid in unraveling the underpinnings of SCA36.
Pioneer SCA Translational Award
The Pioneer SCA Translational Research Award is offered for a research project that will facilitate the development of treatments for Spinocerebellar Ataxia Type 3/Machado Joseph Disease.
Click the carrot next to the name of the study to see its summary.
Sokol Todi, PhD
Wayne State University
Detroit, MI
Targeting Hsc70-4 to mitigate SCA3/MJD
Summary:
The most common dominantly inherited ataxia in the world is Spinocerebellar Ataxia Type 3, also known as Machado-Joseph Disease. The exact reasons for this disease are unclear and therapeutic options for it in the clinic remain unavailable. Here, we propose to investigate how the protein that causes SCA3/MJD behaves, with special focus on protein-protein interactions that can be targeted in the clinic to inhibit toxicity. The protein at the root of SCA3/MJD is known as ataxin-3. We published recently that neuronal toxicity from pathogenic ataxin-3 is regulated by one of the proteins with which ataxin-3 interacts, a protein folding enzyme known as Hsc70-4. We found that reducing the levels of this enzyme mitigates toxicity caused by pathogenic ataxin-3, and that inhibiting the interaction of pathogenic ataxin-3 with Hsc70-4 also improves phenotype. These data indicate that this partnership is harmful in SCA3/MJD. The objectives of this proposal are to: 1) understand how Hsc70-4 worsens SCA3-like phenotypes, and 2) target this enzyme as a therapeutic entry point for SCA3/MJD.
Young Investigator Award
The Young Investigator Award was created to encourage young clinical and scientific investigators to pursue a career in the field of Ataxia research. It is our hope that Ataxia research will be invigorated by the work of young, talented individuals supported by this award.
Click the carrot next to the name of the study to see its summary.
Giulia Bastianello, PhD
IFOM, Fondazione Istituto FIRC di Oncologia Molecolare
Milano, MI, Italy
Ataxia-Telangiectasia and cytoskeleton stress: pathological implications for neurodegeneration
Summary:
Ataxia-Telangiectasia (A-T; OMIM#208900) is an autosomal recessive disorder that affects many ethnic groups with an incidence that ranges from 1:40,000 to 1:200,000 live births. A-T is the second most common form of ataxia, after Friedreich’s ataxia, that appears in early childhood, and it is caused by null mutations in the ATM (Ataxia-Telangiectasia mutated) gene, an apical kinase involved in the DNA damage response pathway and in the repair of DNA double strand breaks (DSBs). The most prominent symptom of A-T is the progressive cerebellar ataxia, characterized by defects in movement coordination that confines most of the patients to wheelchairs by the age of 10. Additional clinical features of the disease include telangiectasia, immunodeficiency, ionizing radiation sensitivity, cancer predisposition and diabetes. While cerebellar degeneration associated with loss of Purkinje cells is the major neuropathological finding in A-T, other neurological manifestations like peripheral neuropathy and degenerative changes in the basal ganglia can occur at later stages of the disease. The causes of neurodegeneration in A-T are still under debate due to the lack of a proper model system to study the process in vivo. Notably, ATM-knockout rodent and pig models do not recapitulate this phenotype. The ATM protein is predominantly nuclear where it plays a key role in DSB response, implying that DNA damage accumulation is one of the primary feature of A-T. However, ATM is also found in the cytosol, including on neuronal presynaptic vesicles. Upon synaptic stimulation ATM phosphorylates a plethora of non-nuclear proteins, some of which involved in vesicle trafficking. ATM is also localized at peroxisomes responding to oxidative stress in absence of DNA damage and it was shown to mediate an antioxidant response upon DSBs through the regulation of the pentose phosphate pathway. These data highlight the broader role of ATM in the control of cellular homeostasis, which can be particularly relevant in non-dividing cells like neurons where lack of cell cycle progression and DNA replication reduces endogenous sources of DNA damage. It is therefore important to address the functions of ATM in the brain under physiological conditions and what is the source of DNA damage in differentiated neurons. Interestingly, it was shown that neuronal activity induced by glutamate receptors stimulation or membrane depolarization triggers the formation of DSBs, ensuing transcription of immediate early genes; under these conditions ATM is activated and phosphorylates its targets Chk2, Kap1 and ?H2AX. This opens a scenario where unprocessed DSBs and homeostatic functions of ATM could contribute, even sinergistacally, to neuronal cell death under physiological conditions of neuronal activation. In our lab, we have been studying the link between the ATR and ATM DDR kinases and cell mechanics. In particular, my research unveiled a novel function of ATM in controlling cell plasticity by showing that , i) ATM associates to the cytoskeleton and interacts with cytoskeletal regulators; ii) it is activated by uni-axial cell stretching or cytoskeletal stress in absence of DNA damage; iii) lack of ATM affects the plasticity of cells at the cytoplasmic level and compromises their cytoskeletal structure and ability to migrate through constrictions. Several neurodegenerative diseases, including Friedreich’s ataxia, have been linked to cytoskeletal alterations, which can affect microtubules, actin cytoskeleton or neurofilaments. The neuronal cytoskeleton is essential to regulate axonal polarization, outgrowth and stabilization but it also affects axonal transport. Microtubules, motor proteins and, more recently, periodic actin-spectrin rings along the axons, have been implicated in cargo transport. Axonal transport is required to distribute vesicles, organelles and signaling molecules towards the nerve terminals and also to clear mis-folded proteins to avoid toxic aggregates; deficits in axonal transport contribute to the pathogenesis of neurodegenerative diseases. Our goal is to investigate how ATM dysfunction affects the cytoskeletal structures of human neurons, including cerebellar Purkinje neurons, before and after induction of neuronal activity, and the impact of cytoskeletal stress on organelles transport along axons. The work will be performed taking advantage of induced pluripotent stem cells (iPSC) derived from healthy donor and A-T patients, which will be further differentiated into neurons or Purkinje cells. The results of this study will expand our knowledge on the mechanisms behind A-T neurodegeneration.
David Buchholz, PhD
The Rockefeller University
New York, NY
Molecular Mechanisms of Human Purkinje Cell Degeneration in Ataxia-Telangiectasia
Summary:
One of the main difficulties in studying human disease is having a model system that reflects changes seen in human patients. Often model systems such as mice are not affected by diseases and genetic disorders in the same way as humans. Induced pluripotent stem cells (iPSCs) provide a model system to study actual human cells derived from patients. In this technique, a biopsy is taken from patients with a genetic disorder to isolate cells. These cells are then converted into iPSCs which subsequently have the ability to generate any cell type in the human body. In the proposed research we will use iPSCs to model a specific genetic form of cerebellar ataxia, called ataxia-telangiectasia (A-T). Ataxia-telangiectasia (A-T) is a genetic disorder caused by mutations in the ATM gene and characterized by cerebellar degeneration, telangiectasia, increased susceptibility to cancer, and immunodeficiency. A-T affects ~1:40,000-1:100,000 live births worldwide and there is no cure. It has been particularly difficult to study the cerebellar aspects of the disease because mouse models do not show the same deficits as human patients. Within the cerebellum, the Purkinje cell (PC) is highly affected, resulting in dysfunction and cell death. The critical question is therefore what are the molecular differences between human and mouse PCs that cause PCs to die in humans but not in mice? In proposed research, I will create a model system using iPSCs from A-T patients to study human cerebellar Purkinje cells (PCs) affected by A-T for the first time. I will focus on defining molecular differences in gene expression and protein profiles of patients with A-T compared to unaffected, control family members. Finally, I will test new approaches for therapies and create a platform for future drug screening.
Sónia Duarte, PhD
Center for Neuroscience and Cell Biology (CNCB)
Coimbra, Portugal
MicroRNA-specific small molecule modifiers as a new and promising therapeutic approach for Machado-Joseph disease/spinocerebellar ataxia type 3 (MJD/SCA3)
Summary:
Machado-Joseph disease/spinocerebellar ataxia type 3 (MJD/SCA3) is the most prevalent autosomal dominantly-inherited spinocerebellar ataxia in the world. Neurodegeneration in MJD occurs in selective brain regions resulting in a wide spectrum of clinical symptoms such as postural instability, ataxia and oculomotor impairments. Clinical manifestations are severe in MJD patients leading to premature death. Unfortunately, there is still no treatment available. Transcriptional dysregulation and particularly dysregulation of microRNAs (miRNAs), which are small molecules of non-coding RNAs that negatively regulate mRNAs expression, has been shown to play a role in MJD pathogenesis. However knowledge on this subject and in the possibility of using miRNAs as a therapeutic strategy is still very scarce. In a previous work, we demonstrated that delivery of let-7 miRNA to the striatum of a lentiviral (LV) mouse model of MJD was sufficient to reduce neuronal protein aggregation in the context of significantly upregulated LC3-II levels, thus indicating that let-7-mediated autophagy activation is beneficial to MJD. In addition, very recently our group identified mir-9, mir-181a and mir-494 predicted to target ATXN3 3’UTR. Overexpression of these miRNAs, which are abnormally downregulated in MJD models, effectively reduced mutATXN3 levels and ameliorated the associated MJD neuropathology. These achievements demonstrate that it is of crucial importance to pursue with the investigation of miRNA pathway involvement in MJD. Although miRNA-based drugs have still not be approved by FDA for medical intervention, some candidates are currently in phases 1/2 of clinical trials or in clinical development. However, there are some FDA-approved drugs capable of modulating miRNA levels (miRNA small molecule modifiers). Enoxacin, being fluoro-quinolone derivative, belongs to a family of approved antibiotic drugs and was shown to induce a general upregulation of miRNA population. In fact, an in vivo administration of enoxacin efficiently increased the levels of miRNAs in the brain, ameliorating the behavioral phenotype in a rat model of depression characterized by decreased miRNA expression. Moreover, this small drug received an orphan designation for amyotrophic lateral sclerosis (ALS) treatment by the European Medicine Agency (EU/3/15/1459). Enoxacin was also shown to increase miR-34a and miR-504 levels found to be in ALS-iPSCs and capable of reversing miRNAs down-regulation in the SOD1 G93A mouse model of ALS, being beneficial for neuromuscular function in these mice. In other study, Nahar et al. have shown that quinazoline-based small molecules, via the same mechanism as enoxacin, are also able to induce miRNA production. In the contrary, aminoglycoside antibiotics, such as Kanamycin and Streptomycin, have been previously described as inhibitors of Dicer-mediated precursor miRNA (pre-miRNA) processing. Although these molecules have enormous potential, they constitute general activators or inhibitors of miRNAs and a specific miRNA selectively is not possible. However, Deiters’ group have screened 1364 compounds in a cellular assay based on luciferase expression and selective small molecule inhibitors of miRNA-21 and miRNA-122, as well as a selective activator of miRNA-122 have been identified. All these studies confirmed that in fact, miRNAs are high-value “druggable” targets that can also be selectively modulated. Our preliminary data suggest that a set of miRNAs are dysregulated both in a transgenic mouse model of MJD and in neuronal cells derived from iPSCs of MJD patients, which foresees a relevant role for these miRNAs in MJD pathology. Therefore, our aim in this project is to identify promising miRNA-specific small molecules capable of selectively modulate miRNA levels in order to readjust miRNA dysregulated levels in MJD models. We will start by performing a high-throughput analysis to identify miRNA-specific drug activators/inhibitors. Then, we will validate the efficiency of miRNA-specific drug activators/inhibitors in primary cultures of cerebellar granule neurons. Finally, the efficiency of miRNA-specific drugs in modulating miRNAs levels in vivo and their therapeutic potential in MJD will be accessed in a Tg mouse model. Overall, taking advantage of the possibility of modulating miRNA levels using FDA-approved drugs that could function as miRNA-specific drug activators/inhibitors we intend to develop a promising therapeutic approach to MJD, and eventually possible to be applied in other SCAs.
Shane Hellyer, PhD
Monash Institute of Pharmaceutical Sciences
Parkville, Australia
Naturally occurring metabotropic glutamate receptor 1 mutations in rare spinocerebellar ataxias; characterization and pharmacological manipulation
Summary:
Spinocerebellar ataxias (SCAs) are a group of debilitating movement disorders, characterized by abnormal and uncoordinated movement. SCAs are caused by genetic changes in proteins in the brain that are important for normal brain function. One such protein is metabotropic glutamate receptor 1 (mGlu1). mGlu1 has emerged as an exciting new target for the treatment of certain types of SCA, based on genetic differences seen in certain patients that cause a change in mGlu1 function. However, the full contribution of abnormal mGlu1 function to SCA progression is unknown. In addition, there exist a number of drug-like compounds that have the potential to restore normal mGlu1 function in a safe and effective way, but their effects on SCA-associated abnormal mGlu1 proteins have not been fully explored. This project aims to fully characterize the function of abnormal mGlu1 protein found in SCAs, as well as the potential utility of mGlu1 as a drug target for treating these disorders. We will explore how known genetic changes in SCA patients affect the function of mGlu1, and whether we can restore normal function using both existing and new chemical compounds. Overall, this project will provide crucial insight into the contributions of mGlu1 to the SCA disease process, and determine the viability of helping SCA patients using new medicines targeted at restoring mGlu1 function.
Chih-Chun Lin, MD, PhD
Columbia University Medical Center
New York, NY
The common mechanism of SCA35 and gluten ataxia
Summary:
Spinocerebellar ataxia type 35 (SCA35) is a recently identified novel genetic ataxia with autosomal dominant inheritance. It is caused by mutations in the gene TGM6. The protein encoded by TGM6, TG6, can remove the amine of peptides, a process called deamidation. Dietary gluten proteins are rich in glutamine, making them substrates for deamidation. Interestingly, an immune-mediated ataxia, gluten ataxia, is associated with autoantibodies against TG6. This suggests that SCA35, a genetic ataxia, shares a mechanism with gluten ataxia, an immune-mediated ataxia. We therefore thought that a treatment option for gluten ataxia, gluten free-diet, can also help patients with SCA35. Indeed, we recently reported a patient with SCA35 who showed clinical improvement after adopting a gluten-free diet. However, the mechanism that TG6 dysfunction leading to cerebellar ataxia is still unclear. A potential target of TG6 is an ion channel called BK channel. Ion channels are proteins that allow ionic currents to pass through the cell membrane, giving rise to the electrical activities of the nervous tissue. We hypothesize that patients with SCA35 and gluten ataxia may be caused by dysfunction of TG6 that leads to aberrant activity of BK channel. This will cause altered electrical activities in the main output neuron of the cerebellum cortex, Purkinje cells, and eventually results in cerebellar ataxia. We propose to investigate our hypothesis with mouse models of SCA35 and gluten ataxia. The findings may help understand the mechanism of SCA35 and help identify therapeutic targets for SCA35.
Post Doc Fellowships
Post-Doctoral Fellowship Awards are to serve as a bridge from post-doctoral positions to junior faculty positions. Recipients have shown a commitment to research in the field of Ataxia.
Click the carrot next to the name of the study to see its summary.
Huijie Feng, PhD
The Children’s Hospital of Philadelphia
Philadelphia, PA
Mechanisms of and therapy for progressive ataxia due to KCNC1 potassium channel mutation
Summary:
Recent advances in molecular genetics has led to the identification of the cause of a wide range of neurological disorders including ataxia. This provides an entry point and potential target for researchers to develop new treatment. However, a gap remains between genetic diagnosis and translation into therapeutics. K+ channels are a type of ion channel, which are essential membrane proteins in all neurons. Even minor disruptions of ion channel function upset the delicate balance between inward and outward ionic currents and can cause abnormal electrical activity and synaptic transmission. This dysfunction of ion channels – or “channelopathy” – is responsible for many human neurological diseases including ataxia. Second only to drugs targeting G protein-coupled receptors, ion channels modulators are an extremely successful drug class which provides support for the proposed approach. Genetic mutations KCNC1 encoding the potassium (K+) channel subunit Kv3.1 are the cause of Myoclonus Epilepsy and Ataxia due to K+ Channel mutation (MEAK, also known as Progressive Myoclonic Epilepsy Type 7 or PME Type 7). Patients with MEAK suffer from progressive ataxia along with myoclonus, tremor, and epilepsy and are wheelchair-bound by adolescence or early adulthood. No effective treatments have been reported to date, for MEAK or for any PME. This proposed study aims to explain the disease pathomechanisms of MEAK in a novel rodent model at both cellular and behavioral levels, and test the efficacy of a novel Kv3 potassium channel modulator as a potential treatment.
Franziska Pohl, PhD
Washington University in St. Louis
St. Louis, MO
Using a C. elegans model of Spinocerebellar Ataxia 3 to investigate new therapeutic approaches: optogenetic activation of redox signaling in GABAergic neurons activates stress response pathways and extends healthspan
Summary:
Spinocerebellar ataxia type 3 (SCA3), also known as Machado-Joseph disease (MJD), is the most common autosomal dominant inherited SCA. Although the genetic basis of the disease is well established, an expanded polyglutamine (polyQ) tract within the protein ataxin 3 (ATXN3) that leads to protein misfolding, no disease modifying treatments are currently available. Current treatments mainly reduce the symptoms and do not affect the underlying pathophysiology of SCA3. While many disease modifying treatment options have been suggested based on pre-clinical research, including gene silencing, prevention of aggregation, and activation of clearance mechanisms, only a small number have translated to clinical studies, and none have shown strong or impactful efficacy in clinical trials. Thus, there is an urgent need for new insights into disease mechanisms that could form the basis for new treatment approaches. I propose to investigate the role of the mitochondrial unfolded protein response (UPRmit) in preventing and/or clearing unfolded ATXN3 protein aggregates, a potentially novel mechanism underlying SCA3 with the potential for therapeutic intervention. UPRmit has been relatively recently identified as a cellular process that regulates protein folding and aggregation. First identified in the model organism C. elegans (a nematode worm), UPRmit is now emerging as an important cellular process in mammalian systems. Activated UPRmit helps cells and organisms to repair mitochondrial dysfunction by improving protein folding, rejuvenating the proteome, and restoring homeostasis. Since protein homeostasis is challenged due to ATXN3 aggregation in SCA3, I hypothesis that activation of the UPRmit may be beneficial for SCA3. Consistent with my hypothesis, activation of UPRmit has previously been shown to be beneficial in disease models of other neurodegenerative disease. The strengths of C. elegans as an experimental system have enabled the discovery of totally new cellular processes, such as UPRmit, and therefore this system has the potential to provide paradigm-shifting treatments for SCA3. I propose to utilize a C. elegans ataxia disease model that I have worked with previously. This AT3q130 strain expresses a polyQ expanded ATXN3 protein that aggregates, just as the protein does in humans. This AT3q130 strain mimics the SCA3 disease behaviorally as well, with decreased movement that can be scored quantitatively. Notably, the AT3q130 strain has previously been used to identify citalopram, an antidepressant, as a potential SCA3 therapeutic that improved movement and decreased protein aggregation. Notably, citalopram treatment was efficacious in mice, where it improved motor balance and coordination and also decreased the percentage of cells containing ATXN3-positive inclusions. Thus, while C. elegans is a simple model organism with only 959 cells, it has a track record finding unexpected insights relevant to mammalian disease models including SCA3. My preliminary studies show that KillerRed, an ‘optogenetic’ protein that produces reactive oxygen species (ROS) in response to light, can activate beneficial stress response pathways in C. elegans including the UPRmit. Surprisingly, when KillerRed is expressed only in GABA neurons, it is able to activate UPRmit throughout the rest of the organism. This is not true when KillerRed is expressed in cholinergic neurons, suggesting it is a unique underlying biology of GABA neurons that somehow regulates protein homeostasis in the rest of the organism; I propose that this discovery can be used to help with SCA3. Animals expressing KillerRed in GABAergic neurons also have an increased lifespan, which might indicate a better general health of the worms. Since aging is known to influence the ATXN3 phenotype in humans, this lifespan extension also predicts that GABAergic biology I propose to investigate may have beneficial effects for SCA3 treatment. Overall, this study will be the first to investigate the role of UPRmit, ROS, and GABA neurons in SCA3. These studies will expand our understanding of the molecular biology and treatment of SCA3, and potentially other ataxias, by leveraging C. elegans to investigate entirely new paradigms in SCA3. In the long term, I plan to continue leveraging the power of C. elegans to uncover novel biological insights to ameliorate ataxias and neurodegenerative diseases.
Setsuki Tsukagoshi, PhD
University of Florida
Gainesville, FL
RAN proteins in sporadic ataxias and SCA8: diagnostic tools and therapeutic potential of metformin
Summary:
Spinocerebellar ataxia type 8 (SCA8), a slowly progressive ataxia with reduced penetrance, is caused by a CTG/CAG repeat expansion in the ATXN8 gene. SCA8 typically begins in adulthood, and most SCA8 patients first present with gait abnormalities and progress to involve hand incoordination, motor speech disorder (dysarthria), and eye movement abnormalities (nystagmus). Additional non-cerebellar features affect some SCA8 patients, with the most common symptoms including sensory loss, cognitive abnormalities, and hyperreflexia. SCA8 patients typically show cerebellar atrophy and thinning of the molecular layer and loss of Purkinje and granule cells in the cerebellum. The pathogenic mechanisms of the disease are not fully understood, but a mechanism called RNA and protein gain-of-function, in which RNA and protein caused by expanded repeats, are associated with the disease. Repeat-associated non-AUG (RAN) translation, a process by which expansion repeats produce proteins from multiple reading frames in the absence of AUG or near-cognate AUG initiation codons, was originally discovered in SCA8. While RAN translation has now been reported in more than 10 different repeat expansion disorders, the underlying mechanisms and pathways that lead to increases in RAN proteins are not fully understood.Given the prevalence of RAN proteins in expansion diseases, our hypothesis is that these protein play a central role in pathogenesis and also can be used to identify repeat expansions in genetically unknown sporadic ataxias. The Ranum lab has demonstrated that RAN translation is highly regulated by the double-stranded RNA-dependent protein kinase (PKR) pathway such that structured expansion RNAs activate PKR leading to increased expression of RAN proteins. They have also demonstrated metformin, a widely available FDA-approved drug of diabetes mellitus, inhibits PKR in C9orf72 amyotrophic lateral sclerosis (C9-ALS) transgenic mice, decreases RAN proteins, and improves behavior and pathology. Metformin has also shown benefit in several other repeat expansion diseases including Huntington’s disease and myotonic dystrophy. Because SCA8 and C9-ALS are both repeat expansion diseases that express RAN proteins, we believe the promising data that metformin reduces RAN proteins and improves phenotypes in C9-ALS mice makes metformin an exciting candidate drug to test in SCA8. To test this hypothesis I will use the SCA8 mouse model previously generated by the Ranum lab. SCA8 mice develop a fully penetrant SCA8 disease with an early-onset motor phenotype and premature death ranging from 4-18 months. As early as 8 weeks of age, a significant reduction in the ambulatory distance, ambulatory time, and a significant increase in resting time can be detected in these mice by open field analysis and gait analysis. These mice show accumulation of RAN polySer proteins in affected brain regions, including the cerebellum and brainstem. The SCA8 RAN polySer shows a distinct distribution pattern in the white matter and accumulation of this protein increases with age and disease progression in SCA8 BAC expansion mice. This mouse model is an excellent candidate to test the therapeutic potential of metformin in SCA8. To test therapeutic the therapeutic efficacy of metformin, SCA8 BAC transgenic mice and their non-transgenic (NT) littermates will be treated with or without metformin. Mice with and without metformin treatment will be evaluated for differences in behavior, survival and neurodegenerative and histological abnormalities including the levels of SCA8 RAN proteins. These data taken with proposed research aims to show that targeting PKR by use of metformin is a promising therapeutic strategy for SCA8 and other expansion diseases.
Diverse Scientists in Ataxia Predoctoral Fellowship
Diverse Scientists in Ataxia Predoctoral Fellowships are intended to enhance research and/or clinical training of promising students who are matriculated in pre-doctoral or clinical health professional degree training programs and who intend careers as scientists, physician-scientists or other clinician-scientists aimed at continuing Ataxia basic science, translational science or clinical research towards the goal of serving individuals with Ataxia.
Click the carrot next to the name of the study to see its summary.
Felicia Williams, PhD Candidate
Scaglione Lab
Durham, NC
Development of a Positive Selection High Throughput Genetic Screen in Dictyostelium discoideum
Summary:
A number of Spinocerebellar Ataxias (SCAs) including SCA1, SCA2, SCA3, SCA6, SCA7, and SCA17 are caused by presence of an expanded tract of the amino acid glutamine in specific proteins. In these diseases, these long polyglutamine tracts cause proteins to clump up and disrupt brain cell function, ultimately resulting in neurodegeneration. Therefore, one potential way to treat these diseases is to prevent this protein clumping phenomenon. Researchers in this laboratory previously realized that an amoeba naturally had thousands of polyglutamine tracts and later found that this organism is naturally resistant to this polyglutamine protein clumping. They next wanted to identify how this amoeba resists protein clumping. To streamline this process, the authors of this paper have developed a new method to identify genes that suppress protein clumping in this amoeba. Using this method, they hope to identify how nature has prevented protein clumping. This information will then be used to aid in developing therapies to treat diseases including SCAs.
Thank You Donors!
Your contributions allow us to offer these crucial grants that accelerate the development of treatments and search for a cure.
Thank You Volunteers!
Our grant application reviewers are all experts in Ataxia science. Their time and dedication to the Ataxia community help us make sure that quality research studies are selected for funding.
View Prior Year's Funded Research
Read Our Latest Research Blogs
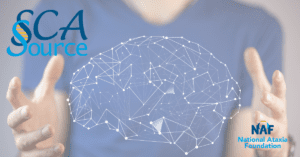
Stay Informed About Ataxia Research with SCAsource
2025 NAF Research Grants
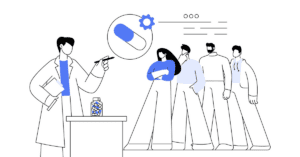
Eligibility in Clinical Trials: Learn More about Inclusion and Exclusion Criteria
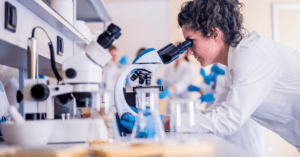
2024 NAF Research Grants
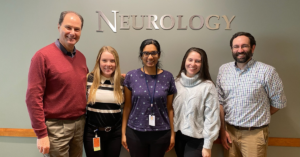
Celebrating a Milestone in Ataxia Research and Community Collaboration
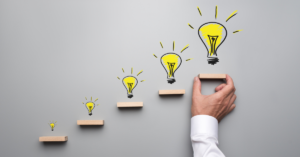