Written by Dr. Sriram Jayabal Edited by Dr. Brenda Toscano-Marquez
Scientists uncover SACS, a gene containing the largest exon identified in vertebrates, which leads to ARSACS when mutated.
What is your morning routine? Coffee first, right? Now, try to think of all the diverse movements you need to make to accomplish this routine. For instance, just to get a cup of coffee, you have to complete a sequence of motor tasks: you start by pulling the pot out of the machine, then you walk to the tap, fill the pot with water, walk back, pour the water into the machine, put your coffee in, and then finally turn on the machine.
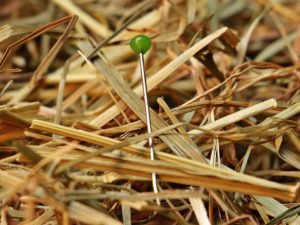
To perform any of these movements, your brain needs to communicate with dozens of muscles in your body. Unfortunately, in people who are affected by hereditary ataxias, the brain loses the ability to coordinate these precise movements. These diseases primarily affect the way patients walk (the symptom that defines “ataxia”), eventually forcing them to use a wheelchair for the rest of their lives.
Hereditary ataxias can be broadly classified as either dominant or recessive. Dominantly-inherited ataxias can be passed down even if only one of the parents is affected; therefore, the disease does not skip generations. Recessive ataxias are inherited from parents who are both carriers of the disease mutation (and who do not usually show any symptoms). Therefore, recessive ataxias can skip generations.
One such recessive ataxia is called Autosomal Recessive Spastic Ataxia of Charlevoix-Saguenay (ARSACS). It was first discovered in people from the Charlevoix-Saguenay-Lac-Saint-Jean region of Quebec, Canada [1]. In this region, it is estimated that one out of every 22 individuals is a carrier for the disease mutation [2]. Though prevalent in this specific area of Canada, ARSACS has now been identified all across the world. Symptoms usually start in early childhood when toddlers are learning to walk. These children experience stiffness in the legs (spasticity) and incoordination in their gait (ataxia), leading them to fall more often. They also have difficulties writing, speaking, and performing tasks that require manual dexterity (usually actions that involve hand movements, like reaching for and grasping an object). They continue to experience worsening gait as they age, often needing a cane or handrail to move around by the time they reach adolescence. Around this time, many patients also experience retinal hypermyelination (an eye abnormality) and peripheral neuropathy (damage to the nerves throughout the body). By their thirties, they become dependent on a wheelchair. There is currently no cure for ARSACS, so it is imperative to study this disease’s underlying causes to identify effective treatments.
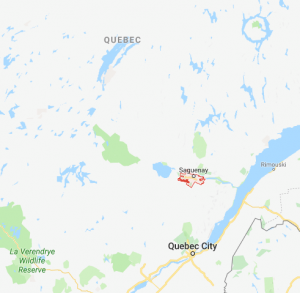
With any disease, however, effective treatment depends on a correct diagnosis. There are more than 40 dominantly-inherited ataxias [3] and more than 10 recessive ataxias [4]. Considering all these possible disease subtypes, one might ask: how are patients diagnosed with a specific ataxia? As it turns out, this is a multi-step process. First, a clinician will make a prediction of the patient’s ataxia subtype after careful consideration of all their symptoms (known as a “differential diagnosis”). Second, a group of clinicians and scientists predict the gene that might be mutated based on what is currently known about the suspected ataxia’s genetic cause. Lastly, a genetic test is conducted to confirm the mutation in the predicted gene, acting as a final verification of the diagnosis of that patient’s ataxia subtype. However, this begs the question: what happens if the predicted mutation is determined to not be the cause of the patient’s ataxia? In this case, a wider genetic screen that tests for other potential ataxias and their associated mutations is needed. In the end, the precision and strength of a diagnosis often depends on the information available in the literature. For instance, when people in the Charlevoix-Saguenay region of Quebec started showing ataxia symptoms in the 1970s, the information available at that time caused them to be diagnosed with Friedreich’s ataxia (another recessive ataxia). This was later classified as ARSACS, a separate disease caused by a mutation in a different gene. As shown in cases like this, it is essential to continuously update the literature to help scientists and clinicians make the correct diagnosis.
In 2000, Engert and colleagues made an important addition to this literature by identifying the gene responsible for ARSACS. What’s more, they even took it a step further: they cloned the gene! This is a difficult task, since the human genome, which is a person’s complete genetic make-up, consists of 3 billion base pairs (the single letters of DNA code) that harbor an estimated 21,000+ genes. Looking for the causative gene in a genome is like looking for a needle in a haystack!
When it comes to combing through genes, scientists employ a process roughly similar to what a crime-fighting detective might do to locate a potential suspect. First, the detective identifies the suspect’s general location. Similarly, the first step in identifying the gene responsible for a genetic disease involves locating the causative gene at the level of chromosomes. In a previous study, Engert and colleagues determined that the gene responsible for ARSACS in somewhere on chromosome 13 [5]. The second step involves triangulating the location of the suspect to a particular area. In this study, by analyzing and comparing the genomes of 53 ARSACS patients and carriers, the candidate region responsible for ARSACS was narrowed down to one small portion of chromosome 13. To identify the genes that are present in this region of the chromosome, they turned to molecular analysis (a close examination of the DNA and other molecules derived from DNA), where they eventually identified the SACS gene by trial-and-error. Think of this as a detective narrowing down their pool of potential suspects based on alibis. The researchers found that the SACS gene consisted of the single largest exon (the DNA that encodes an actual piece of a protein, making it the most meaningful type of code within a gene) ever discovered in vertebrates. Now that the identity of the primary suspect was known, the team needed hard evidence to convict. To validate if the identified gene contributed to ARSACS, they sequenced the DNA from ARSACS patients and unaffected individuals and found a frameshift deletion mutation and a nonsense mutation in the giant exon. Both of these types of mutations lead to the production of an aberrant protein (a protein that functions abnormally), suggesting that the SACS gene is indeed mutated in ARSACS. Despite convicting their suspect, the details of the crime – that is, the ways in which this gene contributes to ARSACS – were still a mystery.
To shed light on this, the process by which the SACS gene produces a protein (known as the gene’s “expression”) was studied further. Researchers found that the SACS gene is expressed in a wide variety of tissues, including the brain, uterus, kidney, and liver. Upon evaluating the characteristics of the protein produced from the SACS gene, known as Sacsin, it was predicted that this protein helps fold other proteins into their normal, functional shape. In addition, they identified the corresponding SACS gene in mice, which was mapped to chromosome 1. This final step is crucial to speed up the discovery of the underlying causes of ARSACS, as animal models of the disease can now be created by mutating a mouse’s SACS gene.
Since the discovery of the SACS gene in 2000, over 170 patient mutations have been mapped to it, which has helped expand the clinical spectrum and diagnosis of the disease [6]. Furthermore, a mouse model of ARSACS was generated, which recapitulated the symptoms seen in humans. In humans, ARSACS affects different brain regions, including the primary motor coordination center of the central nervous system (the cerebellum or “little brain”). Recently, using an ARSACS mouse model, researchers have identified physiological deficits in the cells of the cerebellum [7] – cells that are known to be affected in many other ataxias. With further research using animal models, it’s possible that an effective therapy for this disease could be designed. After clinical trials, we might then be able to treat ARSACS in patients, allowing them to continue their morning routine with minimal intervention.
Key Terms
Recessive Inheritance:A condition that requires two copies of a mutated gene to manifest.
Gene: A unit of heredity made up of DNA that fully or partially controls the development of specific traits.
Conflict of Interest Statement
The author and editor declare no conflict of interest to declare,
Citation of Article Reviewed
Engert, J.C., et al., ARSACS, a spastic ataxia common in northeastern Quebec, is caused by mutations in a new gene encoding an 11.5-kb ORF. Nature Genetics, 2000. 24: p. 120-125. (https://www.ncbi.nlm.nih.gov/pubmed/10655055).
References
- Bouchard, J., et al., Autosomal recessive spastic ataxia of Charlevoix-Saguenay. Canadian Journal of neurological sciences, 1978. 5(1): p. 61-69.
- De Braekeleer, M., et al., Genetic epidemiology of autosomal recessive spastic ataxia of Charlevoix‐Saguenay in northeastern Quebec. Genetic epidemiology, 1993. 10(1): p. 17-25.
- Rossi, M., et al., Autosomal dominant cerebellar ataxias: a systematic review of clinical features. European Journal of Neurology, 2014. 21(4): p. 607-615.
- Anheim , M., C. Tranchant , and M. Koenig The Autosomal Recessive Cerebellar Ataxias. New England Journal of Medicine, 2012. 366(7): p. 636-646.
- Engert, J.C., et al., Autosomal recessive spastic ataxia of Charlevoix–Saguenay (ARSACS): high-resolution physical and transcript map of the candidate region in chromosome region 13q11. Genomics, 1999. 62(2): p. 156-164.
- Baets, J., et al., Mutations in SACS cause atypical and late-onset forms of ARSACS. Neurology, 2010. 75(13): p. 1181-1188.
- Ady, V., Toscano-Marquez, B., et al., Altered synaptic and firing properties of cerebellar Purkinje cells in a mouse model of ARSACS. The Journal of physiology, 2018.